Got Choices?
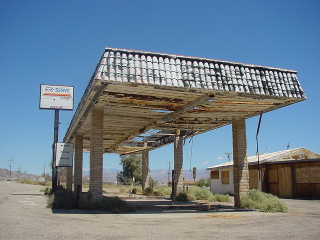
A Future Look at Chemical Energy
Like electrical power distribution, the distribution of chemical energy is highly regulated by government. The primary reason your car is powered by gasoline or diesel fuel, rather than some other compound, may just be that these forms of energy seemed the easiest and cheapest at the time the automobile was initially developed.
Automobile producers, fuel refineries and filling stations have adapted to using commonly defined standards so we can buy a car from the manufacturer of our choice and still fill up with fuel nearly anywhere in the country. In order to assure this standardization, government regulations have evolved to dictate how it will all be done. They determine not only the formulations of the fuels the car will use, but also that the cars will meet certain emission and safety standards. And like most other government regulations, they make it easier for existing big business to continue earning profits, and harder for new, smaller startups to get into the business.
Initially, the fuel distribution infrastructure focused on gasoline, at least where personal automobiles were concerned. But trucks were better suited for operation on diesel fuel and so fuel stations in common trucking corridors catered to both gasoline and diesel. Due to the laws of supply and demand, diesel fuel initially cost less than gasoline even though it provided some improved economies.
Eventually automobile manufacturers caught on to using diesel powered engines to offer these advantages to their customers. As diesel powered cars became more popular, demand for diesel fuel increased and so more and more fuel stations offered it for sale. Now decades later, a new equilibrium has been reached where diesel is typically more expensive than gasoline and diesel powered automobiles are fairly common.
So are these two fuels the only truly viable options for powering a vehicle?
Recent techological advances have finally made electric cars a reality. Perhaps the most notable example is the Tesla, but more are being introduced.
Other cars have been produced to run on Compressed Natural Gas (CNG). Gasoline engines are easily adaptable to operation on this alternate fuel source.
Unfortunately, these alternative systems have their own technical challenges to overcome.
For example, it requires costly energy and an expensive machine to compress the gas into a tank small enough to fit into a car. And it isn’t always easy to find a filling station that offers CNG. At this writing, the number of stations are a tiny fraction of those offering only gasoline and diesel fuel.
Electric cars can be a challenge because they have limited driving range and they can be relatively slow to recharge. They require massive banks of batteries which can be dangerous, caustic to the environment and very expensive when they wear out and have to be replaced.
The primary challenge surrounding the operation of automobiles on alternative storage methods has to do with a concept called energy density. It describes how much energy you are able to fit into a given space or mass.
For example, the energy your car uses is stored in the gas tank. If you have ever looked under your car, you may know about how big that tank is. It probably fits under the trunk or one of the fenders.
You have an idea about how far you can drive on a tank full of gas. And that gives you an intuitive sense of how potent a form of energy gasoline is.
If you buy a car powered by CNG, you will quickly come to realize that your trunk, or truck bed, may be partially or entirely consumed by the tank required to hold the gas. In other words, you have to use up more space in the vehicle just to store fuel. And in spite of this increased space requirement, you may not be able to travel as many miles than you could in your gasoline or diesel powered car.
If you are brave enough to be an early adopter of an electric car, you will likely appreciate the acceleration and efficiency but you may not be able to travel very far on a single charge. The car may also be quite heavy as a result of its massive battery bank. Furthermore, while you can refill your gasoline or CNG car in just a few minutes, it may take much longer to recharge your electric car. These factors make current electric vehicles less practical for longer journeys.
A brief study of energy densities quickly reveals why we like gasoline so much as a storage mechanism for our cars. And make no mistake, gasoline is not really an energy source–it is really a storage medium, much like a battery. The energy itself came from a fusion reaction happening inside the sun and is stored in the molecular bonds of the hydrocarbons in the fuel.
So whether you drive a conventional car or one powered by alternative means like electricity, the power still has to come from somewhere. And most likely it will come from the energy stored in a fossil fuel, at least for a while until we find a more efficient way to get it directly from the sun as it shines. So you may think your new Tesla isn’t polluting the environment in Los Angeles. But somewhere in Nevada, a coal-fired power plant is still polluting the environment as it generates the electrical energy you are using to recharge your batteries.
Gasoline boasts a density of about 34 MJ/L (Million Joules per liter). A Joule is a measure of energy, just like a Kilowatt-hour, but on a much smaller scale. Diesel fuel is around 38 MJ/L. By comparison, natural gas is only 0.03 MJ/L. When we compress natural gas in order to put it in our vehicles, we can raise it all the way up to about 9 MJ/L. But it takes some energy to do that. And 9 is still a lot less than 34. If we can compress it so much that it becomes a liquid, we can get all the way up to about 22 MJ/L. This is still less than 34, and the pressures are too high to efficiently deal with and can be very dangerous.
For reference, a Zinc-air battery can be found in the range of 6 MJ/L. This is still a lot less than 34. Battery technologies and their associated energy densities are constantly increasing. But it looks, for the moment, like the chemical bonds of gasoline and diesel fuel maintain a difficult standard for alternative technologies to compete with.
But are there other ways of storing chemical energy we have not yet thoroughly explored? This is where we might peek into one possible future alternative: elemental carbon (think of coal, but without any of the impurities) has an energy density near 73 MJ/L! Is it any wonder why we rely so heavily on coal for our energy industry?
While most people probably don’t get that excited about the idea of a coal-powered car, let us consider one powered by pure elemental carbon, or graphite, as it is commonly called. When coal is burned, it often emits a number of noxious fumes including sulphur dioxide which, among other bad things, causes acid rain. Graphite however, emits only one thing when burned: pure carbon dioxide.
Now you’re probably thinking “CO2, that’s bad too, right?” Well, not necessarily.
There is a natural energy cycle associated with CO2. Plants consume water and CO2 in the process of photosynthesis to effectively store the energy of sunlight in molecules composed largely of hydrogen and carbon. They throw off the unneeded oxygen into the atmosphere.
Animals consume the oxygen and the vegetation, producing the energy they need to survive, giving off CO2 as a byproduct. That CO2 is then available for the plants to reuse and the energy cycle repeats over and over.
It’s not really a matter of whether oxygen and CO2 are good or bad, but whether a healthy balance in the energy cycle is achieved. There are plenty of polarized opinions about that question but we will not digress by asserting one here. Rather, just consider the following:
What if we could develop a type of solar collector that would act like a plant? It would collect sunlight and use it to split CO2 molecules. But rather than creating hydrocarbons, it would simply output pure carbon, and pure oxygen.
Whereas, CO2 represents a low-energy state, the separated carbon and oxygen represent a much higher energy state. In other words, when we let them recombine through the process of oxidation, we can get that energy back out. And according to our research on energy densities, we could get double or more of what we are getting out of gasoline for the same amount of storage space!
Regardless of your Faith about whether CO2 is bad or good, this process is carbon neutral because the only CO2 it produces is what it has already removed from the atmosphere.
This technology has already been developed in a few early forms. It is theoretically possible to build large collectors which can separate CO2 out of the air. And it is now possible to use an energy source such as sunlight to split that CO2 into its separate constituents of pure carbon and pure oxygen. As this technology progresses, it may become efficient enough to make solar plants that synthetically produce pure carbon.
An alternate approach to carbon production does not try to mimick the way plants can pull CO2 from the air but rather harnesses it. Our environment is filled with abundant sources of carbon such as garbage and biomass that are currently just left to decompose, releasing their CO2 into the air. Through the process of pyrolysis, much of these waste products can be turned into carbon.
Pyrolysis is a well understood reaction that is often used to produce gas fuels such as hydrogen and carbon monoxide. But so far, the carbon byproduct has mostly been suggested for permanent sequestration or use in certain soil remediation. If pure carbon is rich store of energy, why don’t we use it?
For example, could we make a carbon powered car? First, we would have to develop a motor that produces mechanical power from the kind of energy stored in carbon. The traditional internal combustion engine is well suited to liquid fuels, but it could pose potential difficulties for a fuel that is naturally in a powdered or solid form. Perhaps some technologies already exist that could be adapted to this use. A variant of the steam engine would clearly work but may not be very efficient and you would always have to keep filling it with water.
Some proponents claim the Stirling engine would work at the desired efficiencies. And it has the advantage of not using internal combustion. Sometimes the Stirling engine has been called an “external combustion” engine. All it really requires is heat in order to operate. You can use almost any kind of fuel that will burn and it will begin to produce mechanical power.
An alternate approach is to merge carbon fuel technology with the electric car. In 1896, it was discovered how to convert carbon to electricity in a Direct Carbon Fuel Cell. Such technology would bypass the battery problem altogether, allowing you to enjoy the benefits of an electric car without the limited range and slow charging times.
Imagine if these carbon-generating technologies could be implemented on a small enough scale that itcould become a cottage industry. For example, unproductive parcels of land might hold solar powered, carbon collector plants. Other entrepreneurs might create local pyrolysis plants for processing garbage, yard waste and dead trees. Each of these would produce quality, high-density energy carbon for use in cars and trucks.
You could buy it by the bag or pull your fancy new graphite-powered car up to a dispenser and fill it up much the same way as you might at a gasoline filling station. The fuel that powers our cars could be sold informally and safely–without layers of government regulation. You might see a farmer on a corner selling melons, apples and bags of carbon. Not only would your fuel tank take you farther than it used to, but the supply of fuel would be ubiquitous throughout the economy–easy to find and easy to buy.
And because it can all be produced domestically, we can be free from the exploits of foreign oil cartels–free to choose what we put in our fuel tank, free to live and enjoy the full productivity of our own labors.